Terrestrial Vertebrates
Stegocephalians: Tetrapods and other digit-bearing vertebrates
Michel Laurin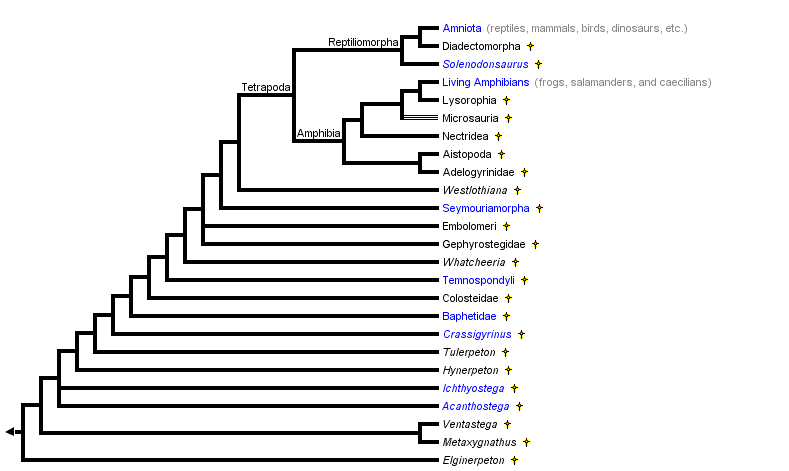


This tree diagram shows the relationships between several groups of organisms.
The root of the current tree connects the organisms featured in this tree to their containing group and the rest of the Tree of Life. The basal branching point in the tree represents the ancestor of the other groups in the tree. This ancestor diversified over time into several descendent subgroups, which are represented as internal nodes and terminal taxa to the right.
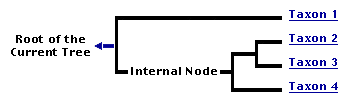
You can click on the root to travel down the Tree of Life all the way to the root of all Life, and you can click on the names of descendent subgroups to travel up the Tree of Life all the way to individual species.
For more information on ToL tree formatting, please see Interpreting the Tree or Classification. To learn more about phylogenetic trees, please visit our Phylogenetic Biology pages.
close boxModified from Carroll (1995), Laurin and Reisz (1997), and Laurin (1998a-c). The position of Whatcheeria follows Lombard and Bolt (1995). The position of the poorly known Devonian taxa (those in which the presence of digits is uncertain: Elginerpeton, Metaxygnathus, Ventastega, and Hynerpeton) follows Ahlberg (1998). Temnospondyls are often thought to be early amphibians. However, notice that in this phylogeny, they are not closely related to lissamphibians.
Introduction
This page discusses terrestrial vertebrates and other vertebrates that bear limbs with digits rather than fins. The muscular limb characteristic of this clade generally has well-defined joints and digits (fingers and toes) and is called a chiridium. This group includes about 21100 extant species and a probably much greater number of extinct species. Tetrapods are only one of the several groups of chiridium-bearing vertebrates (see the section on classification below).
Terrestrial vertebrates have a worldwide distribution. The earliest members of this group were moderately large (1-2.5 m body length). The oldest known skeletal remains of terrestrial vertebrates were found in the Upper Devonian of East Greenland (Clack, 1994). The presence of Lower to Middle Devonian trackways in Australia has led to suggestions that this group may have originated in the Lower Devonian, at least 400 million years ago (Warren et al., 1986), but digits are not visible in these impressions, so these trackways may have been left by other sarcopterygians.
The largest group of terrestrial vertebrates is Tetrapoda (see the section "Classification of Terrestrial Vertebrates", below). Tetrapoda means "four feet", and the group was so-named as its members primitively had four limbs, as opposed to fins. This taxon includes about 3000 extant species of amphibians (frogs, salamanders, and caecilians) and approximately 18100 extant species of amniotes (mammals, reptiles, and birds). The number of extinct species of tetrapods is of course unknown, but about half of the currently known species of tetrapods are extinct (Carroll, 1988).
Tetrapods originated no later than the Mississippian (about 350 million years ago), the period from which the oldest known relatives of living amphibians are known. Relatives of amniotes must have been present at that time, but they have not been found so far. The fossil previously reported (Smithson et al., 1994; Carroll, 1995) as an early Mississippian amniote or anthracosaur (Westlothiana) is probably either a stem-tetrapod or an early amphibian (Laurin, 1998a). Stegocephalians (see section on classification below for a definition of this group) originated no later than the Upper Devonian.
Tetrapods range from 9.8 mm (in the frog Psyllophryne didactyla) to 30 m (in the blue whale) in overall length. They have a worldwide distribution and inhabit all major habitats. Most are terrestrial, but several have returned to the aquatic environment in which our distant ancestors lived. Aquatic tetrapods include various salamanders (sirenids, cryptobranchids, proteids, etc.), frogs (pipids), some caecilians (typhlonectids), leatherback turtles, sea snakes, pinnipeds (seals and walruses), and whales. Some tetrapods are capable of flight (birds and bats), while others glide, such as flying squirrels, dermopterans (sometimes called "flying lemurs", even though they are not primates), and the flying dragons (Draco volans).
The page Life History of stegocephalians contains information on this complex topic.
The main breathing organ of most stegocephalians is the lung, but other respiratory organs exist in many groups. More detail is available on the Breathing in stegocephalians page.
Many stegocephalians have a tympanum for hearing high-frequence, air-borne sounds, and a lateral-line organ is found in many aquatic amphibians. For more information, see the Hearing in stegocephalians page.
Characteristics
Stegocephalians have an extensive fossil record (Carroll, 1988). Phylogenetic studies have revealed several derived characteristics (synapomorphies) of stegocephalians:
- Loss of several cranial bones. In panderichthyids (the group of sarcopterygians most closely related to stegocephalians), the skull was rigidly linked to the shoulder girdle by several bones that disappeared early in the evolution of terrestrial vertebrates. The loss of these bones also allowed the appearance of a mobile neck that allows the head to be moved relative to the trunk. This decoupling allows the head to remain relatively stable while walking.
- Loss of the opercular bones that cover the gill chamber in bony fishes. The operculum was no longer needed in early choanates because they had lost the internal gills of their early ancestors. However, the operculum may have disappeared before the internal gills (Coates and Clack, 1991).
- A reduction of the notochord and a rigid spine. The vertebral centra of osteolepiforms are thin and surround the notochord (a rigid rod present in all chordates and that persists in man as the intervertebral disks) without constricting it greatly. In stegocephalians, the centra are thick and they constrict the notochord. Special articulatory surfaces (the zygapophyses) link the neural arches to each other. A shorter notochord that does not extend into the braincase. The notochord of osteolepiforms extended up to the vicinity of the pituitary.
- Four muscular limbs with discrete digits (fingers and toes). Early finned sarcopterygians such as Eusthenopteron had fleshy fins with elements homologous to the humerus, radius, ulna, intermedium, ulnare, femur, tibia, fibula, and fibulare, but the homology of more distal limb elements is uncertain and controversial. It is presently unclear if the segmented, branched, endoskeletal radials in the distal portion of the fin of sarcopterygians such as Eusthenopteron or Tiktaalik are homologous with digits. Both arguments for (Shubin et al., 2006) and against (Laurin, 2006) this hypothesis have been presented.
- A sacral rib connecting the axial skeleton (the spine) to the pelvic girdle (the hip). This allows the weight of the body of tetrapods to be transmitted to the hind limb. There was no bony connection between the pelvic girdle of osteolepiforms and their axial skeleton. The loss of dermal fin rays (the modified scales that support the fins). This simply represents the elimination of a structure that was no longer needed and may even have been harmful on land.
These characters did not appear simultaneously and suddenly. The oldest known stegocephalians, such as Ichthyostega and Acanthostega, possess intermediate conditions for some of these characters and lack others. For instance, Ichthyostega retained a subopercular, a bone that was part of the opercular complex that covered the gill chamber of osteolepiforms. Acanthostega retained an anocleithrum, which is one of the elements that linked the shoulder girdle to the skull in osteolepiforms (Coates and Clack, 1991). The notochord of Ichthyostega and Acanthostega extended deeply into the braincase, and most of its caudal vertebrae lacked zygapophyses (Jarvik, 1952). The connection between the sacral rib and the pelvic girdle of Acanthostega was still poorly defined. Finally, both Ichthyostega and Acanthostega retain lepidotrichia in the tail, indicating that these taxa still had a caudal fin.
The previous list includes only skeletal characters because all the earliest groups of stegocephalians are extinct, and soft anatomical characters can only be studied in extant taxa. The following characters are found in tetrapods, but not in other extant vertebrates:
- A layer of dead, horny cells that reduces evaporative water loss. This layer is present in amniotes and in most lissamphibians. The keratin helps maintain layers of lipids which reduce water loss (keratin itself has poor water-proofing properties).
- A well-developed muscular tongue with glands. However, some lissamphibians have only a primary tongue, like fishes. A primary tongue is simply a fleshy fold on the floor of the mouth that lacks intrinsic muscles and with limited mobility.
- A parathyroid gland involved in controlling the level of calcium in the blood.
- A Harderian gland located anterior to the eye. This gland secretes an oily liquid that lubricates the eye.
- A vomeronasal (Jacobson's) organ. This olfactory organ is located in the palate and is probably used to smell the food in the mouth.
- The loss of the internal gills. The external gills present in many aquatic and larval lissamphibians are new structures and are not homologous with the internal gills of fishes.
It is difficult to determine exactly when these characters appeared because they are not preserved in fossils, except for indirect clues about the internal gills, and the closest known relatives of tetrapods are extinct. However, these characters are not found in lungfishes (the closest extant relatives of tetrapods). Acanthostega, a Devonian stegocephalian, still had internal gills (Coates and Clack, 1991), and Ichthyostega may have retained them too, but no other stegocephalian is known to have had them. Therefore, internal gills were probably lost early in the evolution of stegocephalians, in the Devonian or the Mississippian (about 360 million years ago), and no tetrapod ever had internal gills.
Classification of Terrestrial Vertebrates
In the past, most terrestrial choanates were included in Tetrapoda (Gaffney, 1979). Recently, Tetrapoda was formally defined as a crown-group (Gauthier et al., 1989). A crown-group is a clade that includes the last common ancestor of two or more extant taxa, and all its descendants. In this case, Tetrapoda was defined as the clade that includes the last common ancestor of lissamphibians and amniotes, and all its descendants.
According to Gauthier et al. (1989), Tetrapoda included most known fossil terrestrial vertebrates because temnospondyls were thought to be the stem-group of amphibians, whereas embolomeres, gephyrostegids, and seymouriamorphs were thought to be more closely related to amniotes than to lissamphibians. Therefore, only a few very early terrestrial vertebrates, such as Ichthyostega and Acanthostega, were excluded from Tetrapoda.
The choanate phylogeny presented here suggests that temnospondyls, embolomeres, gephyrostegids, and seymouriamorphs are not part of the crown-group. If it is accurate, these taxa are not tetrapods and the origin of the "tetrapod limb" predates the origin of Tetrapoda. In the first versions of this page, all sarcopterygians bearing digits were simply called terrestrial vertebrates because no formal phylogenetic taxonomy of this whole clade had been proposed. Such a taxonomy was recently published (Laurin, 1998a). The taxon Stegocephali (that included for a long time all the vertebrates bearing a chiridium, except for lissamphibians and amniotes) was defined as all choanates more closely related to Temnospondyli than to Panderichthys (the closest relative of tetrapods known to have retained paired fins). Therefore, Stegocephali includes all taxa that bear digits, and a few (Elginerpeton, Metaxygnathus, Ventastega, and Hynerpeton) that may retain paired fins. Contrary to the old usage of this term, Stegocephali now refers to a clade. The term stegocephalian will be used below because it does not convey the hypothetical and probably somewhat erroneous interpretation that all digit-bearing vertebrates are terrestrial. As explained below, the earliest members of that clade were probably primitively aquatic.
Note about the node marked Amphibia: Others restrict the name Amphibia to descendents of the most recent common ancestor of extant amphibians (the terminal taxon Living Amphibians in this tree). The author prefers the usage indicated in the tree above, and the definition of Amphibia as all tetrapods more closely related to extant amphibians than to amniotes has historical precedence (Laurin, 1998a), but the second usage has been fairly widespread and cannot be ignored. In this page, the term Amphibia always refers to the node indicated in the tree above, and extant amphibians are referred to as lissamphibians.
Note about the node marked Tetrapoda: Others expand the use of this name to include all vertebrates that possess limbs with digits (Laurin and Anderson, 2004). However, in this page, Tetrapoda always refers to the node indicated in the tree above.
Discussion of Phylogenetic Relationships
The phylogeny of stegocephalians is controversial. Nearly all systematists agree that extant amphibians form a monophyletic group (Lissamphibia) that is closely related to amniotes, but the origin of lissamphibians and amniotes is controversial. Laurin and Reisz (1997, 1999), Laurin (1998a, b), and Vallin and Laurin (2004) suggest that lepospondyls are the stem-group of amphibians, and that diadectomorphs are more closely related to amniotes than to lissamphibians. Consequently, many groups of Paleozoic stegocephalians, such as temnospondyls and seymouriamorphs, are not closely related to amniotes or to lissamphibians:
However, most early studies and a few recent ones have suggested that lissamphibians are derived from temnospondyls, and that diadectomorphs, seymouriamorphs, and embolomeres are more closely related to amniotes than to lissamphibians (Bolt, 1969; Gaffney, 1979; Gauthier et al., 1988, 1989; Milner, 1988, 1993; Panchen and Smithson, 1988; Trueb and Cloutier, 1991; Lombard and Sumida, 1992; Ruta et al., 2003a, b; Ruta and Coates, 2007). Lepospondyls have often been viewed as a paraphyletic group that included early relatives of temnospondyls and lissamphibians. Therefore, most known stegocephalians were viewed as related to either lissamphibians or amniotes. Only Ichthyostega and other Devonian taxa (Acanthostega, Tulerpeton) were not believed to be on one of these two main evolutionary lineages:
A few paleontologists still propose a polyphyletic origin of extant amphibians (Anderson, 2007, 2008; Carroll, 2007; Anderson et al., 2008), with gymnophionans nested within "lepospondyls" and anurans and urodeles nested in temnospondyls. This implies that gymnophionans are more closely related to amniotes than to anurans and urodeles. This phylogeny is incompatible with all published molecular and most morphological phylogenies, which suggest that extant amphibians form a clade which excludes amniotes (Laurin, 2002). Furthermore, reassesment of the coding of the main supporting matrix shows that it actually supports a monophyletic origin of lissamphibians among "lepospondyls" (Marjanovic and Laurin, 2009). Developmental data have also been proposed to support extant amphibian polyphyly (Schoch and Carroll, 2003; Fröbisch et al., 2007), but these arguments have been refuted (Schoch, 2006; Germain and Laurin, 2009; Marjanovic and Laurin, 2009). The developmental data do not discriminate between various hypotheses on lissamphibian origins.
The most recent phylogeny advocating lissamphibian polyphyly (Anderson et al., 2008) is based partly on the recently-described temnospondyl Gerobatrachus hottoni, which was initially interpreted as the sister-group of Batrachia, which is deeply nested in temnospondyls; Gymnophiona is nested in "lepospondyls". That conclusion is based partly on the interpretation of various morphological characters of Gerobatrachus, such as pedicely of the teeth (a lissamphibian synapomorphy), a basal commune (fused distal tarsals 1 and 2; a batrachian synapomorphy), and a narrow, laterally-directed palatine (a salientian synapomorphy). However, the presence of synapomorphies of several nested clades, and of other, much more primitive characters, such as the retention of postparietal, tabular and supratemporal, suggests extensive homoplasy. Other interpretations of the morphology of Gerobatrachus hottoni are possible, and incorporation of that form in the first data matrix which supported lissamphibian polyphyly (McGowan, 2002) still supports a monophyletic origin of Lissamphibia within lepospondyls (Marjanović and Laurin, 2008b).
A few molecular studies have proposed very early dates for the beginning of lissamphibian diversification, ranging from about 337 Ma ago (Zhang et al., 2005) to 362 Ma ago (San Mauro et al., 2005; Roelants et al., 2007). Zhang et al. (2005) also suggested that this iimplied that lissamphibians derived from temnospondyls, but Lee and Anderson (2006) pointed out that such early diversification dates were more compatible with a polyphyletic hypothesis, in which extant amphibians form a clade which excludes amniotes, but derive from both "lepospondyls" and temnospondyls. However, new molecular and paleontological dating of the diversification of lissamphibians suggests a much more recent diversification for this group, in the Permian (Marjanović and Laurin, 2007). Calculation of the confidence interval on the true stratigraphic range of Lissamphibia, based on further development of the most sophisticated method developed by Marshall (1997) also suggests a Permian, monophyletic origin of the group (Marjanović and Laurin, 2008a).
Additional discussion and more detailed phylogenies can be found on the Phylogeny of stegocephalians page.
Origin of Stegocephalians
Several scenarios have been proposed to explain the origin of the chiridium and why vertebrates ventured onto dry land. A theory found in most popular books is that the arid climate that was once thought to have prevailed in the Devonian had forced our sarcopterygian ancestors to crawl out of seasonally drying ponds to reach larger, deeper bodies of water (Romer, 1933). However, a problem with this scenario is that the Devonian is no longer viewed as having been seasonally dry, and classical Late Devonian formations, such as the Escuminac in Quebec, Canada, are now thought to have been estuarine (hence, no seasonal drought could possibly occur, as the sea level is largely independent of seasons). The fin endoskeleton of finned tetrapodomorphs, recently studied in the tristichopterid Eusthenopteron, was apparently very spongy and appears ill-suited to resist significant mechanical stress for extended periods of time, as would have occurred in long terrestrial excursions (Laurin et al., 2007). Furthermore, recent studies on functional morphology suggest that the limbs may have evolved to allow our early ancestors to walk at the bottom of shallow ponds or swamps (Edwards, 1989). Discovery of fairly complete specimens of the Devonian choanate Acanthostega (Coates and Clack, 1990) confirms that its limbs were poorly suited to walk on dry land (Zimmer, 1995). The inferred presence of internal gills in Acanthostega also confirms that this animal was still mainly aquatic (Coates and Clack, 1991). The transition from the aquatic to the terrestrial environment appears to have taken longer than previously thought.
Early Evolution of the Limbs
Elements homologous with the stylopod (femur and humerus) and zeugopod (radius, ulna, tibia, and fibula) have long been known to occur in tetrapodomorphs (e.g. Gregory, 1911), but until recently, little was known about the timing of appearance of various characters of the tetrapod limb. Much progress has been made on this front recently. For instance, a recent histological study has shown that Eusthenopteron foordi paired fins possessed true long bones that grew in length and thickness through endochondral and periosteal ossification, respectively, as in tetrapods (Meunier and Laurin, in press). Boisvert et al. (2008) showed that the distal portion of the pectoral fin of Panderichthys, one of the most crownward finned tetrapodomorphs, is composed of several radials, as should be expected in a form closely related to stegocephalians. The earlier reconstruction that represented a single, large distal plate instead of several smaller radials appears to be a misinterpretation.
Until recently, the oldest unrefutable evidence of the presence of digits was represented by skeletal remains from the very end of the Devonian (Famennian). Four Devonian taxa tentatively included in the tree presented above are known from fragmentary remains. The fragmentary nature of the remains of these genera precludes a confident assessment of their affinities, although Coates (1996) and Ahlberg (1998) have suggested that they are more closely related to tetrapods than to panderichthyids (the closest known relatives of tetrapods known to have retained paired fins). These enigmatic taxa include Elginerpeton (Ahlberg, 1995), Ventastega (Ahlberg et al., 1994), Metaxygnathus (Campbell and Bell, 1977), and Hynerpeton (Daeschler et al., 1994). Their appendicular skeleton is poorly (or not) known, and they are excluded from the smallest clade which includes limbed vertebrates. Therefore, they may have retained fins (Laurin et al., 2000). However, the recent discovery of a Middle Devonian (Eifelian) trackway (Niedzwiedzki et al., 2010) in the abandoned Zachelmie quarry (Poland) makes it more likely that forms such as Elginerpeton possessed digits. Niedzwiedzki et al. (2010) further suggest that this trackway implies that Devonian stegocephalians have a very poor fossil record, to the extent that it implies (in their view) the presence of six long ghost lineages extending from the Middle through the Late Devonian in stegocephalians, in addition to additional ghost lineages in closely related tetrapodomorphs, such as Panderichthy, Tiktaalik, Elpistostege and Livonia (see figure, part A). However, this claim may be exaggerated to the extent that the exact systematic position of the Zachelmie trackmaker is unknown. It could conceivably represent a more basal form, excluded from the smallest clade that includes Elginerpeton, Ventastega and more crownward forms, and this would imply a single stegocephalian ghost lineage extending from the Middle through the Late Devonian, in addition to a few ghost lineages in finned tetrapodomorphs (Laurin, 2010; figure, part B).

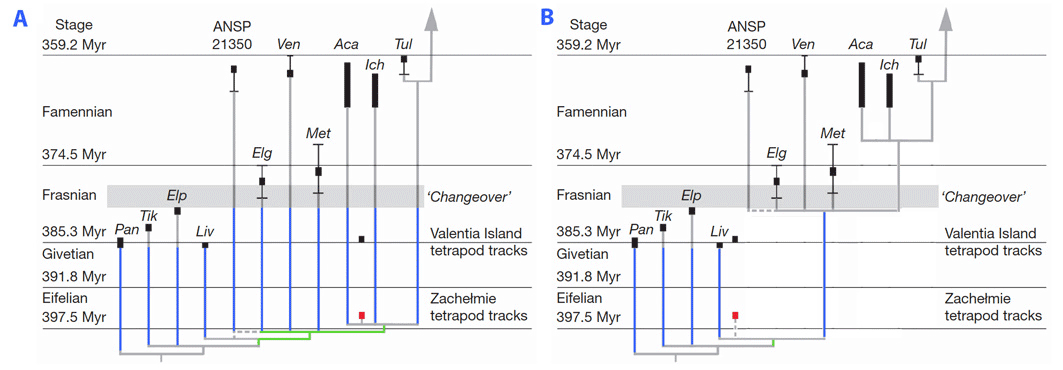
Possible phylogenetic positions of the Polish Middle Devonian Trackway. A, position suggested by Niedzwiedzki et al. (2010). B, positions suggested (in text) by Laurin (2010). Given the available evidence, neither can be refuted. Ghost lineages are in blue. The branches just under the possible position of the trackeway are in green. Part A is redrawn from Figure 5b of Niedzwiedzki et al. (2010).
The presence of five fingers and toes has generally been believed to be primitive for tetrapods and to have been the highest number of digits that our early ancestors had. Five digits were probably present in the earliest tetrapods, but not in the earliest stegocephalians. Recent discoveries have demonstrated that the earliest stegocephalians had more digits; Acanthostega had eight fingers, Ichthyostega had seven toes, and Tulerpeton had six fingers (Lebedev, 1986; Gould, 1991). These "extra" digits were quickly lost, however, because no post-Devonian stegocephalian is known to have had more than five digits.
Coates (1991, 1996) suggested that the pentadactyl condition arose twice (at least in the fore limb) because in his proposed phylogenies, the Devonian polydactylous stegocephalian Tulerpeton was believed to be more closely related to amniotes than to lissamphibians. Therefore, he argued that the reduction from a polydactyl to a pentadactyl condition occurred convergently in anthracosaurs (amniotes and their extinct relatives) and in amphibians (although the pentadactyl condition in amphibians is only know from a few specimens; most lissamphibians have only four digits in the manus). However, reanalysis of these data, and incorporation of Tulerpeton into a data matrix that incorporates more taxa suggests that the pentadactyl condition appeared only once, and well before the divergence between amphibians and anthracosaurs (Laurin, 1998c). This conclusion results from the placement of Tulerpeton in a more basal position in the phylogeny, outside the clade that includes all post-Devonian (and all pentadactyl) taxa.
Conquest of Land by Vertebrates
The first limbed vertebrates, such as Acanthostega and Ichthyostega, were clearly aquatic taxa (Clack, 2002) because they appear to have retained internal gills, as shown by grooves on the ceratobranchials, which may have accommodated afferent branchial arteries. They also retained a postbranchial lamina of the cleithrum, which may have delimited the branchial chamber posteriorly (Coates and Clack, 1991). In addition, they retained lepidotrichia in the caudal fin and a well-developed lateral-line organ, as shown by canals for its cephalic portion.
The internal gills may have disappeared fairly early in the Carboniferous, and there is no trace of lepidotrichia in stegocephalians after the Devonian, and this may indicate a slightly less aquatic lifestyle. However, the lateral-line organ persisted in most Carboniferous and many Permian stegocephalians, which suggests that many species were still primarily aquatic. The absence of grooves for the lateral-line organ is not a reliable criterion to infer a terrestrial lifestyle because the organ may be present without leaving any traces on the skeleton. Since the body shape of early stegocephalians is fairly conservative, aquatic species do not necessarily differ drastically from terrestrial ones in body shape (Laurin, 2008). Thus, additional criteria, such as bone microanatomy, have been studied to determine when vertebrates became terrestrial (Laurin et al., 2004; Germain and Laurin, 2005). Preliminary results suggest that a terrestrial lifestyle appeared in stegocephalians in the Early Carboniferous (Kriloff et al., 2008), but many more species will have to be studied to yield reliable results.
Atmospheric oxygen concentration may conceivably have played a role in enabling vertebrates to move onto land. The first, aquatic stegocephalians appeared while the atmospheric oxygen was present in low concentration. Romer's gap, a period during which very few stegocephalians are known, represents the final phase of this episode of low oxygen concentration. Soon after, as atmospheric oxygen concentration reached and even surpassed its present level, stegocephalians underwent a spectacular evolutionary radiation, which included the first terrestrial vertebrates. That evolutionary radiation was paralleled in arthropods. Thus, Ward et al. (2006) suggest that the oxygen concentration triggered or facilitated terrestrialization in arthropods and vertebrates, and that an oxygen concentration of about 20% in the atmosphere was the lower threshold which allowed a switch from gill breathing in water to air-breathing in vertebrates and arthropods.
Original habitat of stegocephalians
It has long been assumed that the first stegocephalians were freshwater forms, like extant amphibians and dipnoans (Romer, 1933). However, much research from the 1980s onwards has shown that early sarcopterygians (including dipnoans) were most often marine forms (Janvier, 1996). Similarly, several classical Permo-Carboniferous localities that have yielded stegocephalians, and that have long been considered (at least by vertebrate paleontologists) to represent freshwater environments have recently been shown to show marine influence (Laurin and Soler-Gijón, 2006, 2010; Schultze, 2009). It seems likely that many of the earliest stegocephalians were coastal forms, as shown by the Middle Devonian trackways, which were left in an intertidal or lagoonal environment (Niedzwiedzki et al., 2010), and the clearly marine habitat of Tulerperton (Lebedev, 1986). However, some of their close relatives, such as Tiktaalik (Daeschler et al., 2006), and some early stegocephalians, such as Ichthyostega and Acanthostega (Clack, 2002), have been interpreted as freshwater forms. It is likely that stegocephalians inhabited saltwater, freshwater, and terrestrial environments in the late Paleozoic. The intolerance to saltwater that characterizes most lissamphibians appears to be an autapomorpy of Lissamphibia (Laurin and Soler-Gijón, 2010).
References
Ahlberg P. E. 1995. Elginerpeton pancheni and the earliest tetrapod clade. Nature 373: 420-425.
Ahlberg P. E. 1998. Postcranial stem tetrapod remains from the Devonian of Scat Craig, Morayshire, Scotland. Zoological Journal of the Linnean Society 122: 99-141.
Ahlberg P. E., E. Luksevics, & O. Lebedev. 1994. The first tetrapod finds from the Devonian (Upper Famennian) of Latvia. Philosophical Transactions of the Royal Society B 343: 303-328.
Anderson, J. S. 2007 Incorporating ontogeny into the matrix: a phylogenetic evaluation of developmental evidence for the origin of modern amphibians. In: Major transition in vertebrate evolution, (Anderson, J. S. & Sues, H.-D., eds.). pp. 182-227. Indiana University Press, Bloomington.
Anderson J. S. 2008. The origin(s) of modern amphibians. Evolutionary Biology 35: 231–247.
Anderson J. S., R. R. Reisz, D. Scott, N. B. Fröbisch, & S. S. Sumida. 2008. A stem batrachian from the Early Permian of Texas and the origin of frogs and salamanders. Nature 453: 515–518.
Boisvert C. A., E. Mark-Kurik, & P. E. Ahlberg. 2008. The pectoral fin of Panderichthys and the origin of digits. Nature 456: 636–638.
Bolt, J. R. 1969. Lissamphibian Origins: Possible Protolissamphibian from the Lower Permian of Oklahoma. Science 166: 888-891.
Campbell K. S. W. & M. W. Bell. 1977. A primitive amphibian from the Late Devonian of New South Wales. Alcheringa 1: 369381.
Carroll, R. L. 1988. Vertebrate Paleontology and Evolution. New York: W. H. Freeman and Company.
Carroll, R. L. 1995. Problems of the phylogenetic analysis of Paleozoic choanates. Bulletin du Muséum national d'Histoire naturelle de Paris 4ème série 17: 389-445.
Carroll, R. L. 2007. The Palaeozoic ancestry of salamanders, frogs and caecilians. Zool. J. Linn. Soc. 150: 1–140.
Clack, J. A. 1994. Earliest known tetrapod braincase and the evolution of the stapes and fenestra ovalis. Nature 369: 392-394.
Clack J. A. 2002. Gaining ground: the origin and evolution of tetrapods. Bloomington: Indiana University Press, 369 pp.
Coates M. 1991. New palaeontological contributions to limb ontogeny and phylogeny. In: J. R. Hinchcliffe (ed.) Developmental Patterning of the Vertebrate Limb 325-337. New York: Plenum Press.
Coates M. I. 1996. The Devonian tetrapod Acanthostega gunnari Jarvik: postcranial anatomy, basal tetrapod interrelationships and patterns of skeletal evolution. Transactions of the Royal Society of Edinburgh 87: 363-421.
Coates, M. I. & J. A. Clack. 1990. Polydactyly in the earliest known tetrapod limbs. Nature 347: 66-69.
Coates, M. I. & J. A. Clack. 1991. Fish-like gills and breathing in the earliest known tetrapod. Nature 352: 234-236.
Daeschler E. B., N. H. Shubin, K. S. Thomson, & W. W. Amaral. 1994. A Devonian tetrapod from North America. Science 265: 639-642.
Daeschler E. B., N. H. Shubin, & F. A. Jenkins, Jr. 2006. A Devonian tetrapod-like fish and the evolution of the tetrapod body plan. Nature 440: 757-763.
Edwards, J. 1989. Two perspectives on the evolution of the tetrapod limb. The American Zoologist 29: 235-254.
Fröbisch N. B., R. L. Carroll, & R. M. Schoch. 2007. Limb ossification in the Paleozoic branchiosaurid Apateon (Temnospondyli) and the early evolution of preaxial dominance in tetrapod limb development. Evolution & Development 9: 69-75.
Gaffney, E. S. 1979. Tetrapod monophyly: a phylogenetic analysis. Bulletin of the Carnegie Museum of Natural History 13: 92-105.
Gauthier, J., A. G. Kluge, & T. Rowe. 1988. The early evolution of the Amniota. In M. J. Benton (ed.) The phylogeny and classification of the tetrapods, Volume 1: amphibians, reptiles, birds: 103-155. Oxford: Clarendon Press.
Gauthier, J., D. C. Cannatella, K. De Queiroz, A. G. Kluge, & T. Rowe. 1989. Tetrapod phylogeny. In B. Fernholm, K. Bremer, and H. Jornvall (eds.) The Hierarchy of Life: 337-353. New York: Elsevier Science Publishers B. V. (Biomedical Division).
Germain D. & M. Laurin. 2005. Microanatomy of the radius and lifestyle in amniotes (Vertebrata, Tetrapoda). Zoologica Scripta 34: 335-350.
Germain D. & M. Laurin. 2009. Evolution of ossification sequences in salamanders and urodele origins assessed through event-pairing and new methods. Evolution & Development 11: 170–190.
Gould, S. J. Eight (or fewer) little piggies. Natural History, January 1991, 22-29.
Gregory W. K. 1911. The limbs of Eryops and the origin of paired limbs from fins. Science 33: 848-849.
Janvier P. 1996. Early vertebrates. Oxford monographs on geology and geophysics, Oxford: Oxford University Press, 393 pp.
Jarvik, E. 1952. On the fish-like tail in the ichthyostegid stegocephalians with descriptions of a new stegocephalian and a new crossopterygian from the Upper Devonian of East Greenland. Meddelelser om Grønland 114: 1-90.
Kriloff A., D. Germain, A. Canoville, P. Vincent, M. Sache, & M. Laurin. 2008. Evolution of bone microanatomy of the tetrapod tibia and its use in palaeobiological inference. Journal of Evolutionary Biology 21: 807–826.
Laurin M. 1998a. The importance of global parsimony and historical bias in understanding tetrapod evolution. Part I-systematics, middle ear evolution, and jaw suspension. Annales des Sciences Naturelles, Zoologie, Paris, 13e Série 19: 1-42.
Laurin M. 1998b. The importance of global parsimony and historical bias in understanding tetrapod evolution. Part II-vertebral centrum, costal ventilation, and paedomorphosis. Annales des Sciences Naturelles, Zoologie, Paris, 13e Série 19: 99-114.
Laurin M. 1998c. A reevaluation of the origin of pentadactyly. Evolution 52: 1476-1482.
Laurin, M. 2002. Tetrapod phylogeny, amphibian origins, and the definition of the name Tetrapoda. Syst. Biol. 51: 364-369.
Laurin, M. 2006. Scanty evidence and changing opinions about evolving appendages. Zoologica Scr. 35: 667-668.
Laurin M. 2008. Systématique, paléontologie et biologie évolutive moderne : l’exemple de la sortie des eaux des vertébrés. Collection Parcours LMD – Sciences de la vie et de la Terre, ed. J. Segarra. Paris: Ellipses, 176 pp.
Laurin M. 2010. How Vertebrates Left the Water. Translated by M. Laurin. Berkeley: University of California Press, xv + 199 pp.
Laurin M. & J. S. Anderson. 2004. Meaning of the name Tetrapoda in the scientific literature: an exchange. Systematic Biology 53: 68-80.
Laurin, M. & R. R. Reisz. 1997. A new perspective on tetrapod phylogeny. In S. Sumida and K. Martin (eds.) Amniotes Origins: Completing the Transition to Land: 9-59. London: Academic Press.
Laurin M. & R. R. Reisz. 1999. A new study of Solenodonsaurus janenschi, and a reconsideration of amniote origins and stegocephalian evolution. Canadian Journal of Earth Sciences 36: 1239-1255.
Laurin, M., Girondot, M. & de Ricqlès, A. 2000. Early tetrapod evolution. Trends Ecol. Evol. 15: 118-123.
Laurin M., M. Girondot, & M.-M. Loth. 2004. The evolution of long bone microanatomy and lifestyle in lissamphibians. Paleobiology 30: 589-613.
Laurin M. & R. Soler-Gijón. 2006. The oldest known stegocephalian (Sarcopterygii: Temnospondyli) from Spain. Journal of Vertebrate Paleontology 26: 284-299.
Laurin M. & R. Soler-Gijón. 2010. Osmotic tolerance and habitat of early stegocephalians: indirect evidence from parsimony, taphonomy, paleobiogeography, physiology and morphology. In: M. Vecoli and G. Clément (ed.) The Terrestrialization Process: Modelling Complex Interactions at the Biosphere-Geosphere Interface (339): 151–179. London: The Geological Society of London.
Lebedev, O. A. 1986. The first record of a Devonian tetrapod in the USSR. Doklady-Earth science sections 278: 220-222.
Lee, M. S. Y. & Anderson, J. S. 2006. Molecular clocks and the origin(s) of modern amphibians. Mol. Phyl. Evol. 40: 635-639.
Lombard, R. E. & J. R. Bolt. 1995. A new primitive tetrapod, Whatcheeria deltae, from the Lower Carboniferous of Iowa. Palaeontology 38: 471-494.
Lombard, R. E. & S. S. Sumida. 1992. Recent progress in understanding early tetrapods. The American Zoologist 32: 609-622.
Marjanović, D. & Laurin, M. 2007. Fossils, molecules, divergence times, and the origin of lissamphibians. Syst. Biol. 56: 369-388.
Marjanović D. & M. Laurin. 2008a. Assessing confidence intervals for stratigraphic ranges of higher taxa: the case of Lissamphibia. Acta Palaeontologica Polonica 53: 413–432.
Marjanović D. & M. Laurin. 2008b. A reevaluation of the evidence supporting an unorthodox hypothesis on the origin of extant amphibians. Contributions to Zoology 77: 149–199.
Marjanović D. & M. Laurin. 2009. The origin(s) of modern amphibians: a commentary. Evolutionary Biology 36: 336–338.
Marshall C. R. 1997. Confidence intervals on stratigraphic ranges with nonrandom distributions of fossil horizons. Paleobiology 23: 165-173.
McGowan, G. J. 2002. Albanerpetontid amphibians from the Lower Cretaceous of Spain and Italy: a description and reconsideration of their systematics. Zool. J. Linn. Soc. 135: 1-32.
Meunier F. J. & M. Laurin. in press. A microanatomical and histological study of the fin long bones of the Devonian sarcopterygian Eusthenopteron foordi. Acta Zoologica 19 pp., 3 figs, 1 table (Early View version available on the journal's web site).
Milner, A. R. 1988. The relationships and origin of living amphibians. In M. J. Benton (ed.) The Phylogeny and Classification of the Tetrapods, Volume 1: Amphibians, Reptiles, Birds: 59-102. Oxford: Clarendon Press.
Milner, A. R. 1993. The Paleozoic relatives of lissamphibians. Herpetological Monographs 7: 8-27.
Niedzwiedzki G., P. Szrek, K. Narkiewicz, M. Narkiewicz, & P. E. Ahlberg. 2010. Tetrapod trackways from the early Middle Devonian period of Poland. Nature 463: 43–48.
Nussbaum, R. A. 1977. Rhinatrematidae: A new family of caecilians (Amphibia: Gymnophiona). Occasional papers of the Museum of Zoology, University of Michigan 682: 1-30.
Panchen, A. L. & T. R. Smithson. 1988. The relationships of the earliest tetrapods. In M. J. Benton (ed.) The Phylogeny and Classification of the Tetrapods, Volume 1: Amphibians, Reptiles, Birds: 1-32. Oxford: Clarendon Press.
Roelants, K., Gower, D. J., Wilkinson, M., Loader, S. P., Biju, S. D., Guillaume, K., Moriau, L. & Bossuyt, F. 2007. Global patterns of diversification in the history of modern amphibians. Proc. Natl. Acad. Sci. U.S.A. 104: 887-892.
Romer, A. S. 1933. Vertebrate Paleontology. 1st ed., Chicago: University of Chicago Press.
Ruta, M. & Coates, M. I. 2007. Dates, nodes and character conflict: addressing the lissamphibian origin problem. J. Syst. Paleontol. 5: 69-122.
Ruta M., M. I. Coates, & D. D. L. Quicke. 2003. Early tetrapod relationships revisited. Biological Reviews of the Cambridge Philosophical Society 78: 251-345.
Ruta M., J. E. Jeffery, & M. I. Coates. 2003. A supertree of early tetrapods. Proceedings of the Royal Society of London, Series B 270: 2507-2516.
San Mauro, D., Vences, M., Alcobendas, M., Zardoya, R. & Meyer, A. 2005. Initial diversification of living amphibians predated the breakup of Pangaea. Am. Nat. 165: 590-599.
Schoch R. R. 2006. Skull ontogeny: developmental patterns of fishes conserved across major tetrapod clades. Evolution & Development 8: 524-536.
Schoch R. R. & R. L. Carroll. 2003. Ontogenetic evidence for the Paleozoic ancestry of salamanders. Evolution & Development 5: 314-324.
Schultze H.-P. 2009. Interpretation of marine and freshwater paleoenvironments in Permo–Carboniferous deposits. Palaeogeography, Palaeoclimatology, Palaeoecology 281: 126–136.
Shubin, N. H., Daeschler, E. B. & Jenkins, F. A., Jr. 2006. The pectoral fin of Tiktaalik roseae and the origin of the tetrapod limb. Nature 440: 764-771.
Smithson, T. R., R. L. Carroll, A. L. Panchen, & S. M. Andrews. 1994. Westlothiana lizziae from the Viséan of East Kirkton, West Lothian,Scotland, and the amniote stem. Transactions of the Royal Society of Edinburgh 84: 383-412.
Trueb, L. & R. Cloutier. 1991. A phylogenetic investigation of the inter- and intrarelationships of the Lissamphibia (Amphibia: Temnospondyli). In H.-P. Schultze and L. Trueb (eds.) Origins of the higher groups of tetrapods-Controversy and Consensus: 223-313. Ithaca: Comstock Publishing Associates.
Vallin G. & M. Laurin. 2004. Cranial morphology and affinities of Microbrachis, and a reappraisal of the phylogeny and lifestyle of the first amphibians. Journal of Vertebrate Paleontology 24: 56-72.
Ward P., C. Labandeira, M. Laurin, & R. Berner. 2006. Confirmation of Romer’s Gap as a low oxygen interval constraining the timing of initial arthropod and vertebrate terrestrialization. Proceedings of the National Academy of Sciences of the United States of America 103: 16818-16822.
Warren, A., R. Jupp, & B. Bolton 1986. Earliest tetrapod trackway. Alcheringa 10: 183-186.
Zhang, P., Zhou, H., Chen, Y.-Q., Liu, Y.-F. & Qu, L.-H. 2005. Mitogenomic perspectives on the origin and phylogeny of living amphibians. Syst. Biol. 54: 391-400.
Zimmer, C., Coming onto the land. Discover, June 1995, 118-127.
Information on the Internet
For a general introduction to tetrapods and their close relatives, try the Introduction to the Tetrapoda (UCMP, Berkeley). There is an Albanian translation of this page courtesy of Radka Maric, a Chinese translation by Austin Cole from MattressMozz, a Malay translation by Lynly Loh from Down To Five, a Russian translation by UKEdubirdie, a Spanish translation by emfurn.com, and an Ukrainian translation completed by TheWordPoint.Title Illustrations

Scientific Name |
Acanthostega gunnari Ichthyostega |
---|---|
Comments | The Devonian terrestrial choanates Acanthostega (bottom) and Ichthyostega (on the shore). |
Reference | first published in Discover Magazine |
Creator | Illustration by Alfred Kamajian |
Specimen Condition | Fossil -- Period: Devonian |
Copyright | © 1995 Alfred Kamajian |
About This Page
I wish to thank Mr. John Hutchinson, Ms. Patricia Lai, and Mr. Matthew Marlowe who edited this page. I am indebted to Dr. David Maddison who provided invaluable help in formatting this page, in linking it with other pages on the Tree of Life, and whose numerous suggestions improved the presentation of this page. I also thank Katja Schulz for checking my periodic updates before posting them.
Michel Laurin
Muséum National d'Histoire Naturelle, Paris, France
Correspondence regarding this page should be directed to Michel Laurin at
Page copyright © 2011 Michel Laurin
Page: Tree of Life
Terrestrial Vertebrates. Stegocephalians: Tetrapods and other digit-bearing vertebrates.
Authored by
Michel Laurin.
The TEXT of this page is licensed under the
Creative Commons Attribution License - Version 3.0. Note that images and other media
featured on this page are each governed by their own license, and they may or may not be available
for reuse. Click on an image or a media link to access the media data window, which provides the
relevant licensing information. For the general terms and conditions of ToL material reuse and
redistribution, please see the Tree of Life Copyright
Policies.
- First online 04 January 1996
- Content changed 21 April 2011
Citing this page:
Laurin, Michel. 2011. Terrestrial Vertebrates. Stegocephalians: Tetrapods and other digit-bearing vertebrates. Version 21 April 2011. http://tolweb.org/Terrestrial_Vertebrates/14952/2011.04.21 in The Tree of Life Web Project, http://tolweb.org/