Diatoms
David G. Mann


This tree diagram shows the relationships between several groups of organisms.
The root of the current tree connects the organisms featured in this tree to their containing group and the rest of the Tree of Life. The basal branching point in the tree represents the ancestor of the other groups in the tree. This ancestor diversified over time into several descendent subgroups, which are represented as internal nodes and terminal taxa to the right.
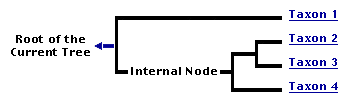
You can click on the root to travel down the Tree of Life all the way to the root of all Life, and you can click on the names of descendent subgroups to travel up the Tree of Life all the way to individual species.
For more information on ToL tree formatting, please see Interpreting the Tree or Classification. To learn more about phylogenetic trees, please visit our Phylogenetic Biology pages.
close boxIntroduction
The diatoms are one of the largest and ecologically most significant groups of organisms on Earth. They are also one of the easiest to recognize, because of their unique cell structure, silicified cell wall and life cycle. They occur almost everywhere that is adequately lit (because most species need light for photosynthesis) and wet - in oceans, lakes and rivers; marshes, fens and bogs; damp moss and rock faces; even on the feathers of some diving birds. Some have been captured by other organisms and live as endosymbionts, e.g. in dinoflagellates and foraminifera. Because of their abundance in marine plankton, especially in nutrient-rich areas of the world's oceans, diatoms probably account for as much as 20% of global photosynthetic fixation of carbon (~ 20 Pg carbon fixed per year: Mann 1999), which is more than all the world's tropical rainforests.

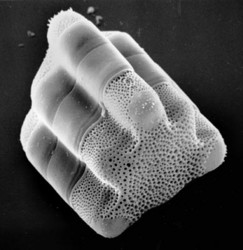
Hydrosera. © David G. Mann. This image comes from the Professor Frank Round Image Archive at the Royal Botanic Garden Edinburgh
Diatom cells have regular geometrical shapes. In a mathematical sense, they are always 'closed generalized cylinders' and they are usually straight ('right') but the cross section of the cylinder can vary from circular to elliptical to spicular to complex lobed shapes like the Hydrosera cell shown above. The shape is maintained faithfully, whatever the environmental conditions, because the cell wall contains a large proportion of hard, brittle silica, which is partially hydrated [(SiO2)m.nH2O] and non-crystalline. Basically, diatoms live in glass boxes. The silica shell of the diatom is called the 'frustule' and is made of two halves, each in turn composed of several different pieces. Hydrosera frustules, like those of all other diatoms, are perforated by many small holes, which allow water, dissolved material and solids (gases, inorganic nutrients, and organic substrates and secretions) to pass in or out.

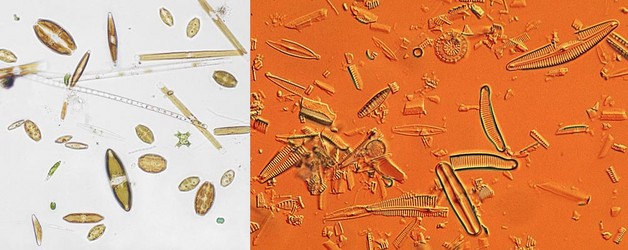
Left: Living diatoms and other algae from a freshwater loch in Scotland. Right: False-colour picture of a subfossil assemblage from a muddy deposit a few metres below the surface of a mire in SW Scotland. © 2008 David G. Mann
The silica of the diatom cell wall is resistant to decay, although it will begin to dissolve once its organic coating has been stripped off. Once incorporated into silica-rich sediments, however, frustules may survive for hundreds to millions of years and can be used to monitor changes in freshwater or marine environments. The left-hand picture above shows a spread of living diatoms and other algae from a freshwater loch in Scotland. Each cell contains one to several brownish chloroplasts. Shown in the right-hand (false-colour) picture is a subfossil assemblage from a muddy deposit a few metres below the surface of a mire in SW Scotland. Here, all the cells are empty - only the cell walls remain; indeed, in many cases the cell walls have fallen apart into their component pieces. But it is still possible to identify them, because the walls retain their shape and pattern. Consequently, if the ecologies of the species are known, then the fossil assemblage can be used to estimate what conditions were like when it was formed. In the assemblage illustrated there are both planktonic species (the circular Cyclotella valves) and benthic species, which have become mixed together after death.


Life cycle series of Navicula reinhardtii valves. © 2008 David G. Mann
Because of the construction of the silica frustule and the way in which cells divide, average cell size declines during the life cycles of most diatoms. The shape often changes too, as in the series of Navicula reinhardtii valves shown. It can take a long time for cells to decline to their smallest size - often several years in nature - but sooner or later there is an abrupt restitution of size, taking a few days, involving formation of a special cell, called an auxospore. This behaviour is unique.
Variation in shape and size during the life cycle causes major problems for people trying to identify diatom species and also for taxonomists, if only a few dead specimens are available for study. If diatoms 'miss' the chance to form auxospores (for example, if suitable mates are not available, or if environmental conditions are unsuitable), the cells continue to divide, getting smaller and smaller until they die.
Characteristics
Diatoms share several characteristics with some or all other heterokont algae, including (see also van den Hoek et al. 1995):
- plastids that are enclosed by four membranes. The inner two are homologous with the two membranes surrounding the plastids of Rhodophyta, Chlorophyta and Glaucophyta. The outer two, often referred to as 'chloroplast endoplasmic reticulum' reflect the origin of the heterokontophyte plastid as a secondary endosymbiont, related to extant Rhodophyta.
- between the outer and inner chloroplast membranes, there is often a network of anastomosing tubules called the periplastidial reticulum.
- grouping of the thylakoids into stacks of three (lamellae) within the plastid.
- presence of a girdle lamella beneath the plastid membranes, surrounding all the other lamellae.
- chlorophylls a and c and fucoxanthin as the major light-harvesting pigments for photosynthesis.
- chloroplast DNA usually concentrated within a ring-shaped nucleoid at the periphery of the plastid (but there are exceptions in some diatoms!)
- a β-1,3-linked glucan as the main reserve polysaccharide.
- possession of special tripartite stiff hairs ('mastigonemes') on a flagellum.
- mitochondrial inner membrane developed into tubular invaginations.
Diatoms share with the bolidophytes a unique 2 amino-acid insertion in the large subunit of Rubisco.
The characteristics of diatoms are that:
- all species are unicellular or colonial coccoid algae. None are free-living flagellates.
- the only flagellate cells produced are the male gametes (= sperm, spermatozoids) of 'centric' diatoms. These have a single forward-pointing flagellum, which bears mastigonemes.
- the relative proportions of the chlorophylls and fucoxanthin produce a yellow-brown or greenish-brown colour in the plastids.
- most have a large central vacuole or pair of vacuoles.
- cells (especially during stationary-phase) often accumulate large quantities of lipids and fatty acids; polyphosphate bodies are also present and sometimes take the form of discrete spherical or complex 'volutin' granules, one per vacuole.
- secretion of extracellular polymeric material (usually polysaccharides) is common, as stalks, pads, capsules, tubes, chitin fibres, or trail material from locomotion.
- all cells (except the gametes and endosymbiotic diatoms) possess a bipartite cell wall comprising two overlapping halves.
- each half-wall itself consists of a large end-piece, the 'valve', and several or many narrow bands or segments, which together form the 'girdle'.
- the cell wall is almost always heavily silicified.
- cell wall elements (valves, girdle bands, and auxospore scales and bands) are formed intracellularly, in special membrane-bound 'silica deposition vesicles' associated very closely with the cell membrane; they are not secreted from the cell until they are complete.
- new wall elements are always produced within the confines of an existing cell wall. As a result, average cell size usually decreases with successive mitotic divisions during the life cycle.
- size is restored via the formation and expansion of a special cell, the auxospore, which is usually a zygote. The basic shape of each diatom species is largely created during the expansion of the auxospore, but is often modified during subsequent mitotic cell divisions.
- during vegetative mitoses, the nucleus always lies to one side of the cell immediately beneath the girdle, at the edge of the hypotheca.
- mitosis is open, the nuclear envelope breaking down before metaphase; the spindle is a narrow cylinder, persistent at telophase, consisting of two interdigitating half-spindles, each associated with a polar plate.
- the chromosomes bunch closely around the cylindrical spindle at metaphase, becoming impossible to separate and count.
- cytokinesis occurs through cleavage.
- the life cycle is strictly diplontic: as far as is known, all vegetative cells of all species are diploid, and all mitoses take place in the diploid phase. However, haploids have occasionally been grown in culture in a few species.
- they occur just about everywhere in aquatic and damp terrestrial habitats, providing that photosynthesis is possible!
- they are amazingly diverse, with hundreds of genera and perhaps 200,000 species (Mann & Droop 1996), of which only a tenth have been described so far.
Relationships of Diatoms to Other Groups
Despite a number of studies to examine phylogeny, using one or several genes, the relationships of diatoms to other groups are still unclear and there is still a huge gap in our understanding of how and when diatoms acquired their unusual morphology and life-cycle characteristics. The diatoms have often been treated as a separate phylum, reflecting their unique features. Pascher (1914, 1921) suggested that the diatoms have features in common with the Chrysophyceae and Xanthophyceae and therefore placed these classes and the Bacillariophyceae in the phylum Chrysophyta. Ultrastructural and molecular sequence data have confirmed the general thrust of Pascher’s idea, placing the diatoms unambiguously among the heterokont protists (‘stramenopiles’) within the chromalveolates (Adl et al. 2005).
In the past, it was sometimes suggested that diatoms evolved well before their appearance in the fossil record and that the early phases in diatom evolution were lost long ago through diagenesis of diatomites to chert (e.g. Round 1981). This is made extremely unlikely by recent molecular phylogenies, which date the origin of diatoms towards the beginning of the Mesozoic Era. Furthermore, a close relationship to other silica scale or silica skeleton-producing algae and protists, such as the Chrysophyceae, is not evident in recent analyses. The closest known relatives of the diatoms are the bolidophytes (Bolidophyceae), which are a small group of marine autotrophic picoplankton with the same kind of plastids and flagellum structure as diatoms and some other autotrophic heterokonts (Guillou et al. 1999). However, bolidophyte cells are highly reduced and simplified and do not seem to produce any silica structures, although it is possible that silicifying life cycle stages have been missed.
Mann and Marchant (1989) suggested that another group, the Parmophyceae, may also be closely related to diatoms and thus may give hints as to how diatoms arose, because they produce silica scales that in some respects (radial pattern subtended by a central ring, space-filling development of pattern) resemble diatom valves and girdle bands. So far, no DNA sequences have been confirmed to be derived from Parmophyceae, but a clade of unknown heterokonts closely related to diatoms and bolidophytes has been detected by Lovejoy et al. (2006) and may represent the Parmophyceae; it is certainly important for understanding the evolution of both bolidophytes and diatoms that the organisms detected by Lovejoy et al. are fully characterized.
Round and Crawford (1981) and Mann and Marchant (1989) developed hypotheses about how the diatom frustule evolved, based on comparative morphology. Both suggested that diatoms probably arose from scaly celled ancestors. The scale-case was thought initially to have been homogeneous (all the scales were fairly alike in size, shape and structure). Then there was a stage in which the scales became differentiated into larger valve-like scales and narrower ones that resembled the segments found in the girdles of modern Rhizosolenia species (though this is not meant to imply that modern rhizosolenids are a basal offshoot), and a still later stage when the proto-girdle bands became even narrower, forming hoops around the cell.
According to this evolutionary progression, valves and girdle bands would have a common origin, which seems reasonable because their structure is often similar and they are formed in similar ways. Furthermore, cells covered evenly with scales are known in diatoms, in the auxospores of some centric diatoms, e.g. Melosira or Ellerbeckia (Crawford 1974, Schmid & Crawford 2001).
The main differences between the Round–Crawford and Mann–Marchant hypotheses are in the assumptions made about the nature of the scales and scaly cell in the early (‘Ur’) diatoms and the nature of the scaly cells themselves. In the Mann–Marchant scheme, the scales of the pre-diatom were space-filling structures, which abutted to form the complete, functional cell wall of a temporarily dormant cyst, whereas Round and Crawford envisaged the scales as separate elements that did not abut but were imbricate, covering growing vegetative cells as in modern synurophytes.
No precursors of diatoms have been identified from the fossil record.
Discussion of Phylogenetic Relationships
Just as it is something of a mystery at present as to how and why the diatoms arose from among the other heterokont algae, so also we have little idea about relationships among the major lineages within the diatoms. At the generic level and, to a lesser extent, within families of diatoms, considerable progress has been made during the last 40 years, as a result of huge injections of new information and analysis, first from electron microscopy (particularly scanning electron microscopy), then from examination of cell structure and sexual reproduction – which are characteristics previously ignored by most diatomists – and most recently from molecular phylogenetics. However, these data have not yet provided a clear idea of higher-level relationships in diatoms, among families, orders and classes. Round et al. (1990) described many new genera and resurrected others from obscurity, on the basis of morphological and cytological surveys; these have on the whole been supported by subsequent investigations, including studies based on molecular data. Round et al. also provided a new framework of classes, orders and families to 'hold' the revised genera. These have not been so successful. Gene sequence data have shown over and over again that informal analysis of relationships, based on morphology, often fails to reveal the true pattern of evolution. The problem seems to be that parallel or convergent evolution of shape and wall structure has been rampant within the diatoms. But in many cases, molecular analyses have also failed us thus far, partly because of convergent evolution and partly because of analytical difficulties, such as establishing homology in rDNA sequences and hence developing a correct alignment matrix.
Round et al. divided the diatoms into three classes: Coscinodiscophyceae, Fragilariophyceae and Bacillariophyceae, which correspond to three of the main types of valve organization. The Coscinodiscophyceae were to be recognized by having valves in which the pattern of ribs and striae (lines of pores) radiates out from a ring (the annulus). In the Fragilariophyceae, the pattern was feather-like, with the ribs and striae being arranged either side of one or two longitudinal ribs or strips (sterna). And in the Bacillariophyceae, the pattern was similar to that in in the Fragilariophyceae, except that the central strip contained a raphe system. Informally, these three structural variants can be referred to as 'centrics' (Coscinodiscophyceae), 'araphid pennates' (Fragilariophyceae) and 'raphid pennates' (Bacillariophyceae).
Although analyses of molecular sequence data have not yet provided us with a clear picture of the early evolution of the diatoms, one thing has become obvious: the Round et al. three-class system is wrong, if the aim is to reflect phylogeny. The phylogenetic trees that have been published during the last 15 years disagree in many respects, but all show the primary radiation to have occurred among diatoms with a centric valve structure, the pennates having evolved later, from ancestors with centric structure. Furthermore, the Fragilariophyceae are not monophyletic, being paraphyletic with respect to the Bacillariophyceae. So, of the three Round et al. classes, only one – the Bacillariophyceae – is satisfactory.
The main features that appear in several phylogenetic trees (once the topologies of the trees have been simplified to include only those relationships that have good statistical support) are:
- a basal polytomy of clades, all but one of which have circular valves (very rare exceptions) with a centric valve pattern and monopolar or radial symmetry; the clades with circular valves and centric organization are often referred to as the 'radial centrics'. The 'radial centrics' may be a monophyletic group and appear so in some published trees; on the whole, however, it appears that they are not.
- a further clade in the basal polytomy that comprises the remainder of the centric diatoms and all of the pennate diatoms. The centric diatoms in this clade usually have a polar organization. The pattern still radiates from an annulus, but the valves are usually elliptical or elongate, triangular or triradiate, etc, and the structure of the valve shows bi- to multipolar symmetry; circular valves are uncommon and possible secondarily derived. Centric diatoms of this kind are often referred to as 'polar centrics'.
- the polar centrics rarely appear in molecular phylogenies as a monophyletic group; instead, they are usually paraphyletic with respect to the pennate diatoms.
- the pennate diatoms are monophyletic.
Medlin & Kaczmarska (2004) proposed a replacement for the Round et al. scheme, based on interpretations of molecular and some nonmolecular data, in which the diatoms were divided into two subphyla, Coscinodiscophytina (= 'radial centrics') and Bacillariophytina ('polar centrics' + pennates), and the Bacillariophytina in turn into two classes, the Mediophyceae ('polar centrics') and Bacillariophyceae (pennates). Note that Medlin & Kaczmarska's use of 'Bacillariophyceae' (all pennate diatoms) differs from that of Round et al (1990) (only raphid pennate diatoms). It is very likely that the Mediophyceae are paraphyletic and quite likely that the Coscinodiscophytina are also paraphyletic. Because of this, and because some published molecular analyses are said not to be repeatable, the Medlin–Kaczmarska scheme has been heavily criticized (e.g. Williams & Kociolek 2007). However, the new classification does have the virtue, relative to the Round et al. (1990) classification, that it reflects better the finding, now generally agreed and consistent with the fossil record, that diversification occurred first among diatoms with a centric valve structure, and that pennates evolved later from within one out of several or many centric clades. Although this is not a new idea (e.g. Simonsen 1979), it was only one of many possibilities aired before the advent of molecular systematics (see Mann & Evans 2007).
Adl et al. (2005) adopted Medlin & Kaczmarska's names but noted that the Coscinodiscophytina and Mediophyceae might be paraphyletic. Here, Medlin & Kaczmarska's formal taxa are used only where there seems to be good support for monophyly (i.e. the Bacillariophytina and Bacillariophyceae). Nevertheless, it is often useful to distinguish Medlin & Kaczmarska's Coscinodiscophytina and Mediophyceae informally, as 'radial centric diatoms' and 'polar centric diatoms', to highlight what seems to be a well-established feature of diatom evolution, that complex shapes and bi- or multipolar structure developed in one clade of centric diatoms (within which the pennates evolved later), but not in several others.
Global Significance
It has been known for a long time that diatoms are abundant in aquatic habitats, forming an essential part of many food chains. However, it was not until the 1990s that their huge contribution to the global carbon economy began to be fully appreciated. A back-of-the-envelope calculation (Mann 1999) goes like this:
- total net primary production for the globe is ~ 105 Pg carbon per year (Field et al. 1998)
- of this, about 46% occurs in the oceans and 54% on land (Field et al. 1998)
- of the oceanic component, about one-quarter (11 Pg) takes place in oligotrophic (nutrient-poor) regions, one-quarter (9.1 Pg) in eutrophic (nutrient-rich) regions, and half (27.4 Pg) in the remaining mesotrophic regions (Field et al. 1998)
- diatoms account for no more than 25-30% of primary production in nutrient-poor waters, but perhaps 75% in nutrient-rich regions (Nelson et al. 1995); so, assume an intermediate value of 50% for mesotrophic waters
- the total contribution made by diatoms is then {(11 × 0.25) + (27.4 × 0.5) + (9.1 × 0.75)} = 23.275 Pg carbon per year, which is ~ 23.5% of the global total
It's probably an overestimate, but the importance of diatoms is evident nonetheless. For comparison, all the world's tropical rainforests fix 17.8 Pg, all the savannas 16.8 Pg, and all the world's cultivated area another 8 Pg. The fate of the carbon that diatoms fix is now a crucial issue in climate-change research.
Another way to appreciate diatoms is to realize that they give us every fifth breath, by the oxygen they liberate during photosynthesis.
General Texts
Round, F.E., Crawford, R.M. & Mann, D.G. (1990). The diatoms. Biology and morphology of the genera. Cambridge University Press, Cambridge. 747 pp.
Stoermer, E.F. & Smol, J.P. (1999). The diatoms. Applications for the environmental and earth sciences. Cambridge University Press, Cambridge. 488 pp.
van den Hoek, C., Mann, D.G., Jahns, H.M. (1995). Algae. An introduction to phycology. Cambridge University Press, Cambridge.
References
Adl, S.M., Simpson, A.G.B., Farmer, M.A., Andersen, R.A., Anderson, R.A., Barta, J., Bowser, S., Brugerolle, G., Fensome, R., Fredericq, S., James, T.Y., Karpov, S., Kugrens, P., Krug, J., Lane, C., Lewis, L.A., Lodge, J., Lynn, D.H., Mann, D.G., McCourt, R.M., Mendoza, L., Moestrup, Ø., Mozeley-Standridge, S.E., Nerad, T.A., Sheraer, C., Spiegel, F. & Taylor, F.J.R. (Max) (2005). The new higher level classification of eukaryotes and taxonomy of protists. Journal of Eukaryotic Microbiology 52: 399-451.
Field, C.B., Behrenfeld, M.J., Randerson, J.T. & Falkowski, P. (1998). Primary production of the biosphere: integrating terrestrial and oceanic components. Science 281: 237-240.
Crawford, R.M. (1974). The auxospore wall of the marine diatom Melosira nummuloides (Dillw.) C. Ag. and related species. British Phycological Journal 9: 9–20.
Guillou, L., Chrétiennot-Dinet, M.-J., Medlin, L. K., Claustre, H., Loiseaux-de Goër, S., Vaulot, D.: Bolidomonas: a new genus with two species belonging to a new algal class, the Bolidophyceae (Heterokonta). Journal of Phycology 35, 368–381 (1999).
Lovejoy, C., Massana, R. & Pedrós-Alió, C. (2006). Diversity and distribution of marine microbial eukaryotes in the Arctic Ocean and adjacent seas. Applied and Environmental Microbiology 72: 3085–3095.
Mann, D.G. (1999). The species concept in diatoms. Phycologia 38: 437-495.
Mann, D.G. & Droop, S.J.M. (1996). Biodiversity, biogeography and conservation of diatoms. Hydrobiologia 336: 19–32.
Mann, D.G. & Evans, K.M. (2007). Molecular genetics and the neglected art of diatomics. In: Unravelling the algae – the past, present and future of algal systematics (Ed. by J. Brodie & J. Lewis), pp. 231-265. CRC Press, Boca Raton, Florida.
Mann, D.G. & Marchant, H. (1989). The origins of the diatom and its life cycle. In J. C. Green, B. S. C. Leadbeater & W. L. Diver (eds.) The chromophyte algae: problems and perspectives (Systematics Association Special Volume 38), pp. 305–321. Clarendon Press, Oxford.
Medlin, L.K. & Kaczmarska, I. (2004). Evolution of the diatoms: V. Morphological and cytological support for the major clades and a taxonomic revision. Phycologia 43: 245–270.
Nelson, D.M., Tréguer, P., Brzezinski, M.A., Leynaert, A. & Quéguiner, B. (1995). Production and dissolution of biogenic silica in the ocean: revised global estimates, comparison with regional data and relationship to biogenic sedimentation. Global Biochemical Cycles 9: 359-372.
Pascher, A. (1914). Über Flagellaten und Algen. Berichte der Deutschen Botanischen Gesellschaft 32: 136–160.
Pascher, A. (1921). Über die Übereinstimmung zwischen den Diatomeen Heterokonten und Chrysomonaden. Berichte der Deutschen Botanischen Gesellschaft 39: 236–248.
Round, F.E. & Crawford, R.M. (1981). The lines of evolution of the Bacillariophyta. I. Origin. Proceedings of the Royal Society of London, B 211: 237–260.
Schmid, A.-M.M. & Crawford, R.M. (2001). Ellerbeckia arenaria (Bacillariophyceae): formation of auxospores and initial cells. European Journal of Phycology 36: 307–320.
Simonsen, R. (1979). The diatom system: ideas on phylogeny. Bacillaria 2: 9–71.
Williams, D.M. & Kociolek, J.P. (2007). Pursuit of a natural classification of diatoms: history, monophyly and the rejection of paraphyletic taxa. European Journal of Phycology 42: 313-319.
Title Illustrations

Scientific Name | Coscinodiscus |
---|---|
Location | Cultured cells from North Sea marine plankton |
Comments | These cells settled into a regular array as a result of slight agitation and vibration of the Petri dish containing them |
Specimen Condition | Live Specimen |
Identified By | David Mann |
Life Cycle Stage | vegetative |
Image Use |
![]() |
Copyright |
© 2008 David G. Mann
![]() |
Scientific Name | Stephanodiscus |
---|---|
Creator | Frank E. Round |
Acknowledgements | This image is derived from the Professor Frank Round Image Archive at the Royal Botanic Garden Edinburgh |
Specimen Condition | Dead Specimen |
Identified By | David Mann |
Life Cycle Stage | Vegetative phase |
Body Part | Valve |
View | External view, SEM |
Image Use |
![]() |
Copyright |
© David G. Mann
![]() |
Scientific Name | Lyrella hennedyi |
---|---|
Comments | Optical focus near one valve; two complex lobed chloroplasts are present, one towards each pole of the cell. |
Specimen Condition | Live Specimen |
Identified By | David Mann |
Life Cycle Stage | Vegetative phase |
Body Part | Single cell |
View | Valve view |
Image Use |
![]() |
Copyright |
© David G. Mann
![]() |
About This Page
This page is being developed as part of the Tree of Life Web Project Protist Diversity Workshop, co-sponsored by the Canadian Institute for Advanced Research (CIFAR) program in Integrated Microbial Biodiversity and the Tula Foundation.
David G. Mann
Royal Botanic Garden Edinburgh, United Kingdom
Correspondence regarding this page should be directed to David G. Mann at
d.mann@rbge.org.uk
Page copyright © 2010 David G. Mann
Page: Tree of Life
Diatoms.
Authored by
David G. Mann.
The TEXT of this page is licensed under the
Creative Commons Attribution-NonCommercial License - Version 3.0. Note that images and other media
featured on this page are each governed by their own license, and they may or may not be available
for reuse. Click on an image or a media link to access the media data window, which provides the
relevant licensing information. For the general terms and conditions of ToL material reuse and
redistribution, please see the Tree of Life Copyright
Policies.
- First online 07 February 2010
Citing this page:
Mann, David G. 2010. Diatoms. Version 07 February 2010 (under construction). http://tolweb.org/Diatoms/21810/2010.02.07 in The Tree of Life Web Project, http://tolweb.org/